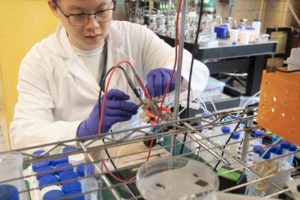
A team from U of T Engineering has invented a device that leverages electrochemistry to increase the efficiency of direct air carbon capture. Their alternative strategy aims to accelerate the widespread adoption of this emerging technology.
“The technology required to pull carbon directly out of the air has been developing for decades, but the field is now accelerating with governments and industry investing in the infrastructure required to actually do this at scale,” says Professor David Sinton (MIE), senior author on a paper published in Joule that outlines the new technique.
“One key barrier is that current processes require a lot of energy, and indeed emit a fair amount of carbon themselves. If we can offer a more efficient strategy, we can make the case to scale this technology to climate-meaningful levels.”
The specific carbon capture technique that Sinton and his team are working to improve is known as a pH swing cycle. It begins when air is pumped through a liquid solution that is strongly alkaline, meaning that it has a high pH. CO2 in the air reacts with the alkaline solution and is captured in the form of carbonates.
To regenerate the capture liquids, chemicals are added to precipitate the carbonates as a solid salt. In the typical process, this salt is heated by burning natural gas to turn the carbonates back into CO2 gas which can be injected underground or upgraded into other carbon-based products.
“If you conduct a life-cycle analysis of this entire process, you see that for every tonne of CO2 you capture, you generate the equivalent of around 300 to 500 kilograms of CO2,” says Yi (Sheldon) Xu (MechE 1T6, PhD 2T0) who worked on the project as a PhD candidate and a postdoctoral fellow in Sinton’s lab. Xu is now at Stanford University.
“You’re still coming out ahead, but the energy inputs, particularly the heating step, cost a lot in terms of overall carbon efficiency.”
To overcome this challenge, the team turned to electrochemistry. Devices known as electrolyzers use electricity to drive forward chemical reactions that would not happen otherwise. Devices known as fuel cells do the opposite, generating electricity from chemical reactions.
The team’s key insight was creating a single device that could operate in both directions, that is, as both a fuel cell and an electrolyzer. This innovation enabled them to open up a new pathway to regenerating the alkaline solutions needed for carbon capture.
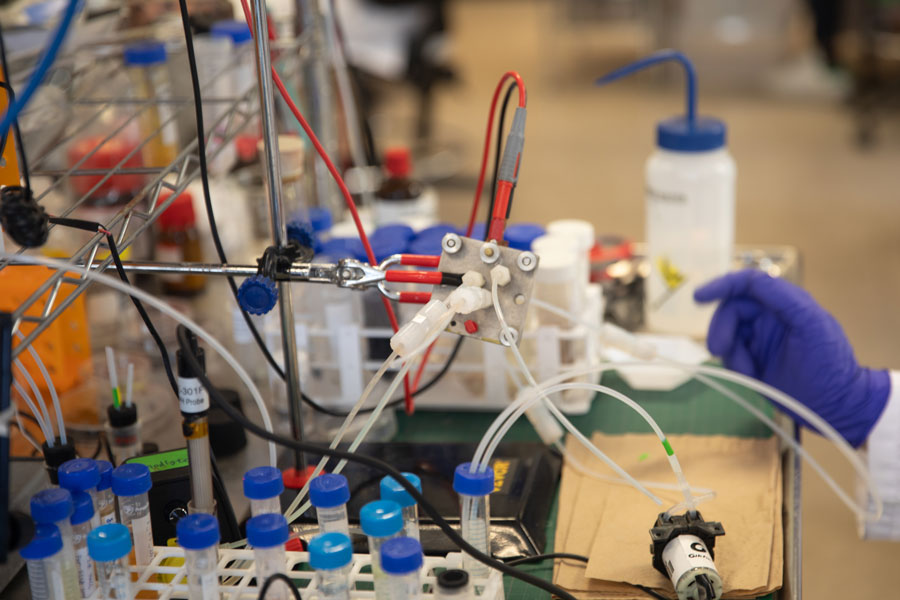
“Both electrolyzers and fuels cells have a positive electrode and a negative electrode,” says Jonathan Edwards (MIE 1T5 + PEY, PhD 2T1), another member of the team.
“In our device, the positive electrode of the fuel cell and the electrolyzer are one and the same. We switch the mode of operation every second, so that two different reactions can happen at the surface of the same electrode.”
In the first of these two reactions, the electrolyzer uses electrical current to extract alkali metal ions and regenerate the strongly alkaline solution needed for air capture. The electrolyzer also produces hydrogen, which is recycled back to the fuel cell side of the device, where it reacts to produce electricity, which in turn is fed back into the electrolyzer.
The fuel cell produces an acidic solution, which is reacted with carbonate salts from the air capture unit to release CO2 gas. After the CO2 is released, the resulting solution is fed back to the electrolyzer, thus completing the cycle.
The process offers several advantages. First, it circumvents the energy-intensive heating step entirely. Second, it uses electricity as opposed to natural gas; this electricity could be obtained from low-carbon sources such as solar, wind or nuclear energy.
Finally, the fact that two reactions happen at a single electrode cuts down on what are known as mass transfer limitations — bottlenecks in how fast the reactants can diffuse to the electrode surface — which increase the amount of energy needed to drive the reaction.
“When we ran the life cycle analysis on our process, we saw that it only generates about 11 kg of CO2 equivalent per tonne of CO2 captured,” says Shijie Liu (MIE PhD candidate), another member of the team.
“That’s about 40 times less than the current thermal process.”
The team has already attracted international interest: as Team E-quester, they placed in the top 60 of the global XPRIZE Carbon Removal held last year. Now that their work has been published, they are hoping that more teams will join them in further optimizing this electrochemical pathway.
“At the moment, we’re focusing on improving the capture fluid and further reducing process energy consumption, ensuring that it’s made of sustainable and low-cost substances, as well as scaling it up to industrial levels,” says Xu.
“But there are other places, such as electrode design, where there could be more innovations to discover. We’d love to see this become a viable new platform for carbon capture plants that are less energy-intensive to build and operate than what we have today. That would give us a powerful new tool to mitigate the impacts of climate change.”
– This story was originally published on the University of Toronto’s Faculty of Applied Science and Engineering News Site on August 2, 2023 by Tyler Irving.