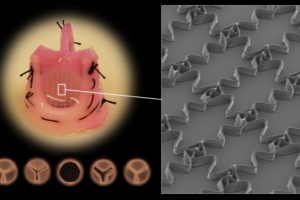
A team of researchers at the University of Toronto, led by Professor Craig Simmons, has introduced a novel method to engineer soft connective tissues with prescribed mechanical properties similar to those of native tissues. This finding, published in the journal Advanced Functional Materials, can propel the generation of more realistic tissues and organs for regenerative medicine in the future.
“Soft connective tissues, including heart valves, possess highly nonlinear and anisotropic mechanical properties that haven’t been accurately replicated in tissue-engineered structures before,” said Bahram Mirani, a PhD candidate and the leading author of the research. “Current tissue-engineered heart valves often fall short of accurately mimicking the intricate mechanical properties of native valves, leading to their eventual failure.”
The research team’s innovative approach combines computational modeling, statistical optimization, and a cutting-edge fabrication method known as Melt Electrowriting (MEW). MEW, a fusion of 3D printing and electrospinning, enables the precise deposition of fine fibers with complex architectures. This method stands out for its ability to create structures with microscopic features that yield native tissue mechanics.
“Melt electrowriting is a powerful biofabrication method to produce intricate fiber architectures. Its ability to precisely print fibers with complex shapes in specific patterns has garnered significant attention in the biomedical field, especially in recent years.” said Mirani.

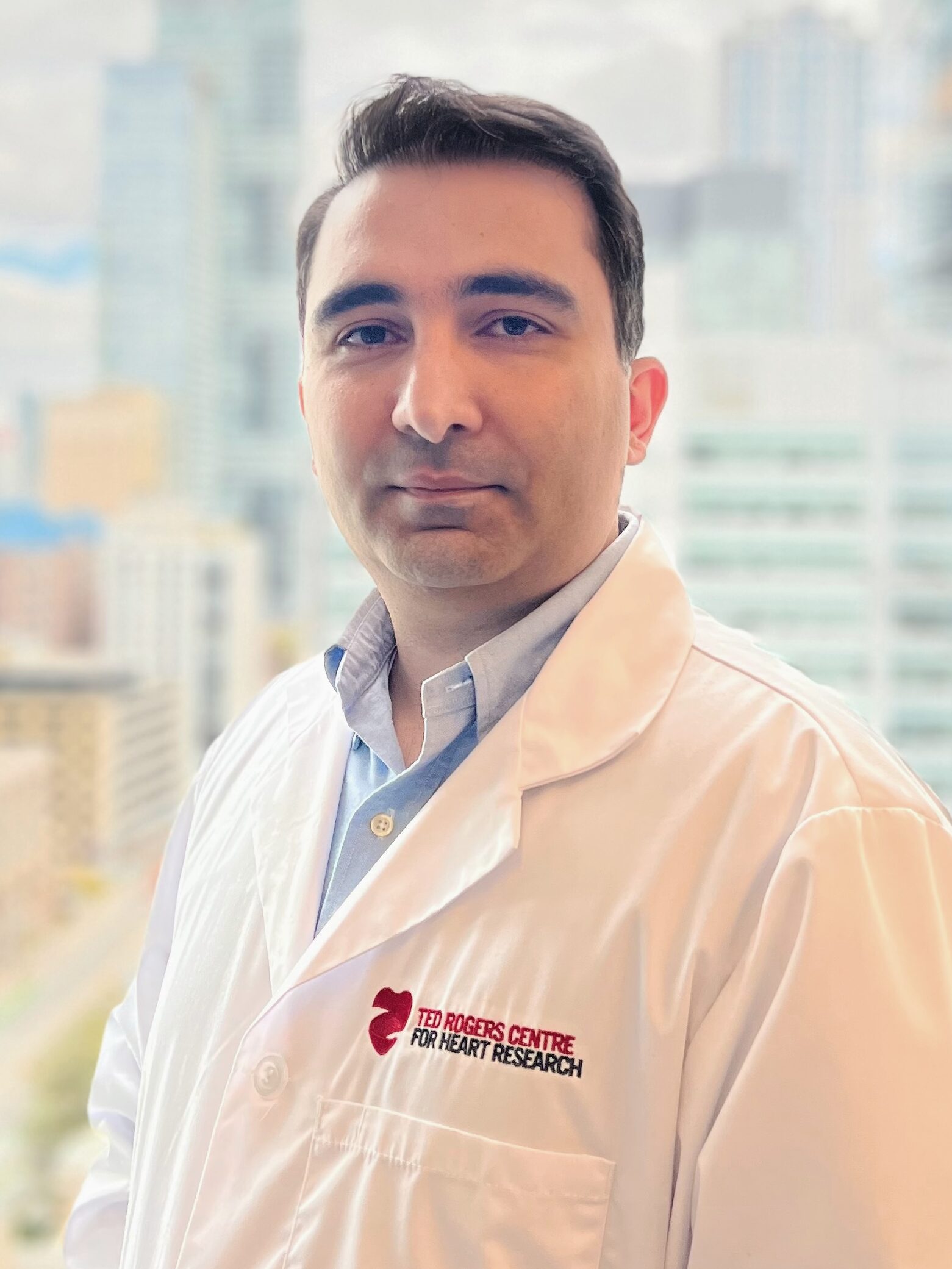
One of the critical features of soft connective tissues is their nonlinearity and anisotropy. Nonlinearity refers to how a tissue stiffens as it is stretched, whereas anisotropy means that the tissue’s stiffness varies in different directions. The MEW method, coupled with computational modeling, enables the replication of these intricate mechanical characteristics.
The computational modeling aspect played a pivotal role in streamlining the optimization process. Mirani elaborated, “Without an optimization method or computational modeling, we would have had to test hundreds of conditions experimentally. Through computational modeling, we reduced the number of experimental conditions needed for optimization down to only five. This significantly accelerated the entire optimization process.”
The research has far-reaching implications beyond cardiovascular applications. Mirani stated, “While our examples focused on heart valve and pericardium tissues, the methodology we’ve developed is applicable to a wide range of tissues and organs with non-linear mechanical properties, such as tendons, ligaments, and skin.”
The ultimate goal of this research is to develop living tissue constructs that can be implanted into patients in the future, such as children with congenital heart conditions. These engineered tissues could grow and remodel alongside the patient, potentially reducing the need for multiple interventions over their lifetime.
“Current treatments for children born with defective heart valves are quite limited. The living replacement heart valves engineered with this new biofabrication approach have unmatched mechanical function, which we expect will contribute to longer-term success than what is possible currently,” said Simmons, the corresponding author of this research.
Collaborators from Queen’s University and the University of Ottawa played crucial roles in the success of this research. The project received funding from various sources, including the Natural Sciences and Engineering Research Council of Canada (NSERC), the Canadian Institutes of Health Research (CIHR), and the Translational Biology and Engineering Program in the Ted Rogers Centre for Heart Research.
This pioneering study opens up new avenues in tissue engineering, promising not only improved clinical outcomes for patients with heart conditions but also paving the way for advancements in various other fields of medicine.
– This story was originally published on the University of Toronto’s Biomedical Engineering News Site on November 15, 2023.